Climate Now Episode 13
July 19, 2021
Bioenergy Conversion with Jerry Tuskan
Featured Experts
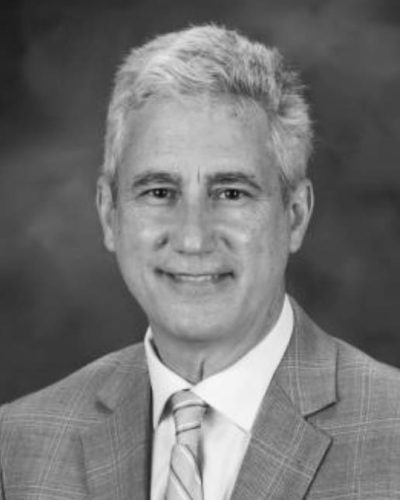
Jerry Tuskan
CEO of the Center for Bioenergy Innovation
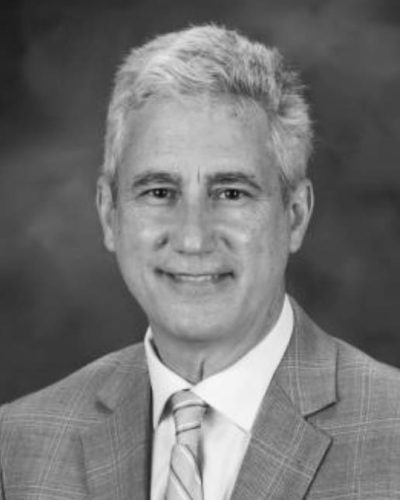
Jerry Tuskan
CEO of the Center for Bioenergy Innovation
Gerald Tuskan is the CEO at the Center for Bioenergy Innovation at Oak Ridge National Laboratory where he researches plant genetics to develop ideal biomass for energy use.
Featured In:
In this Episode
How exactly are plants converted into liquid transportation fuel? And what obstacles does bioenergy need to overcome in order to displace fossil fuels in the US energy economy and abroad?
Jerry Tuskan is the CEO of the Center for Bioenergy Innovation and Group Lead for Plant Systems Biology in the Biosciences Division at Oak Ridge National Laboratory, where he has been since 1990.
He also holds a joint appointment at the Joint Genome Institute – a Department of Energy Office of Science user facility – where he helps lead the Plant Science Program.
Dr. Tuskan joined Climate Now to help us understand the biomass to biofuel process and how the sector can expand to meet our net-zero emissions targets.
Related Media:
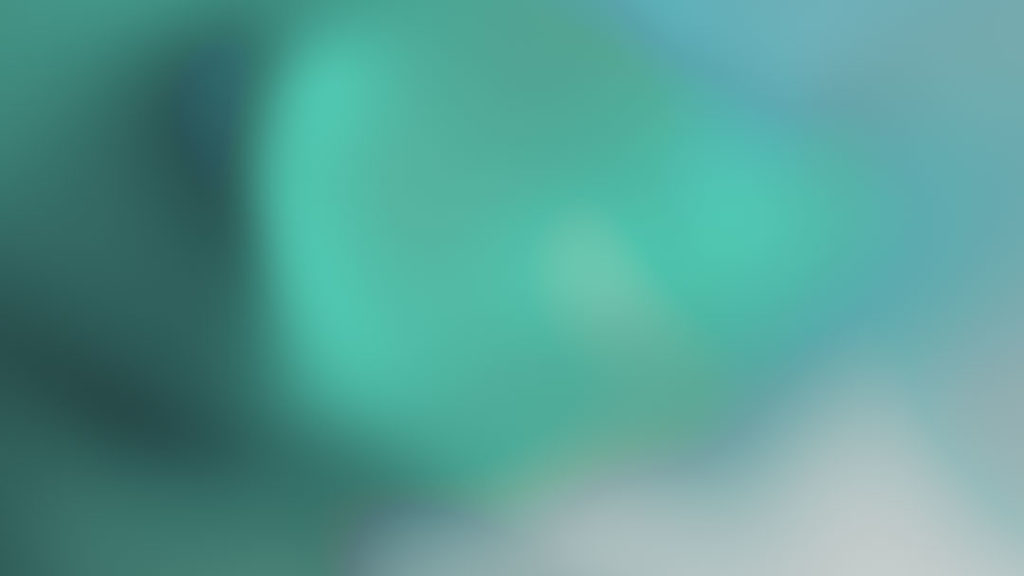
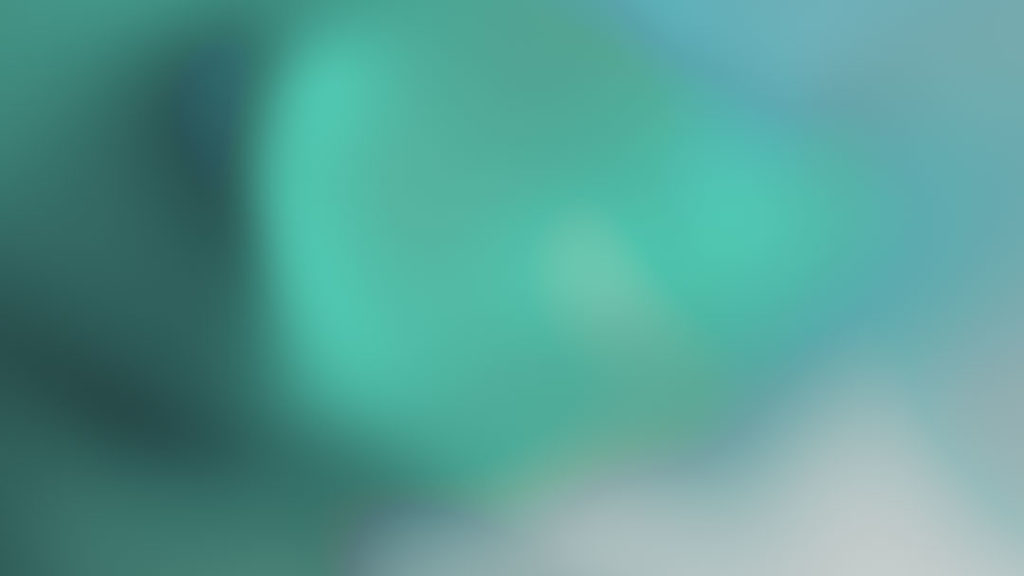
Climate Now: Jul 12, 2021
Biomass Availability with Matthew Langholtz
Bioenergy is a renewable energy for its carbon neutrality – plants absorb CO2 during photosynthesis and emit the same amount when combusted for energy. But to significantly reduce our reliance on fossil fuels, substantial amounts of biomass, or organic m
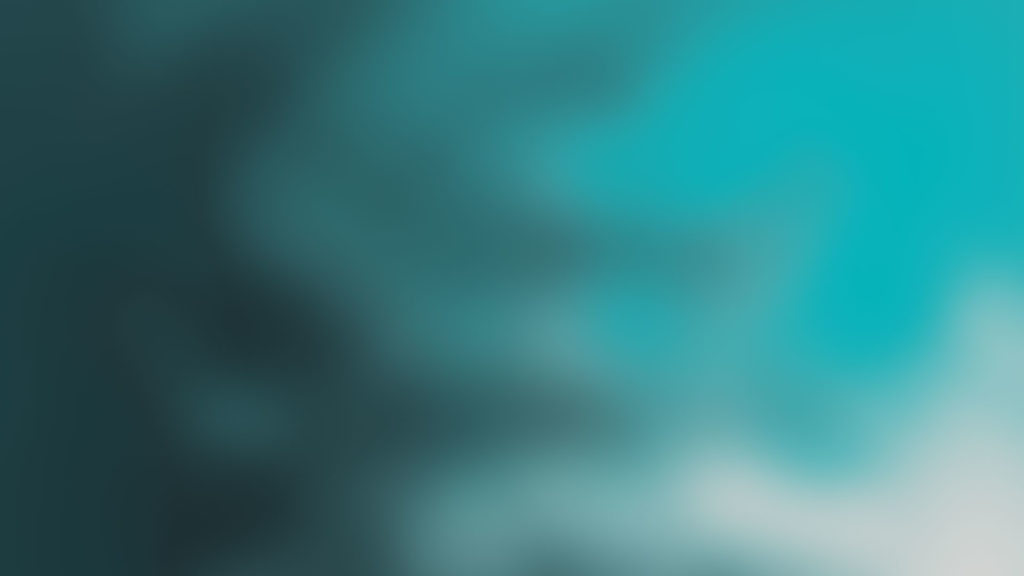
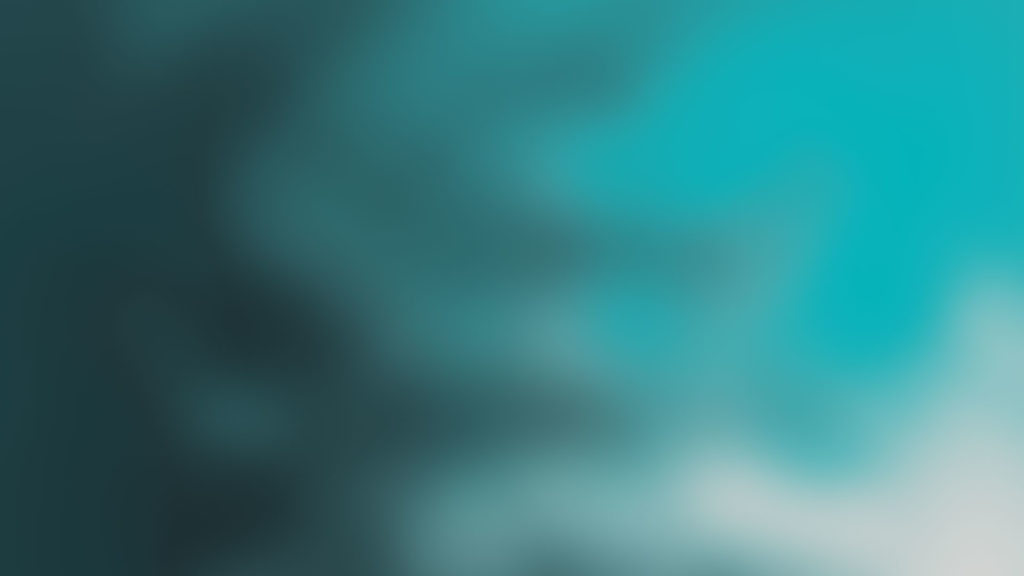
Climate Now: Jun 25, 2021
Net-Zero by 2050 with Eric Larson
What are the possible paths and necessary steps to achieve net-zero emissions in the United States by 2050? Which energy sources could sufficiently decrease our reliance on natural gas and oil to meet that target? And how much will those new energy sources nee
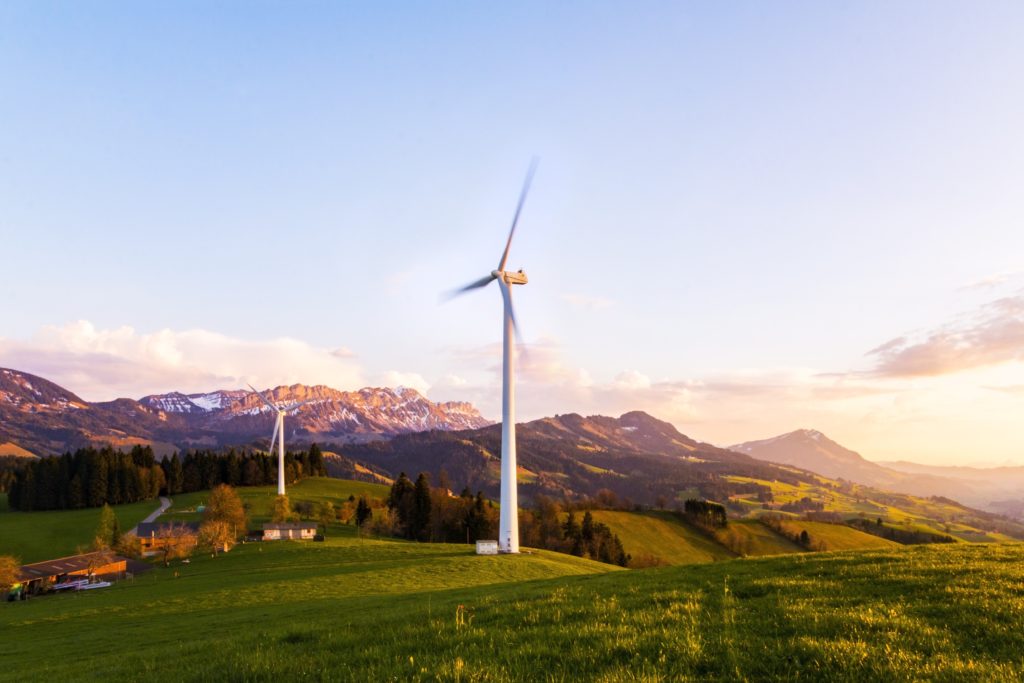
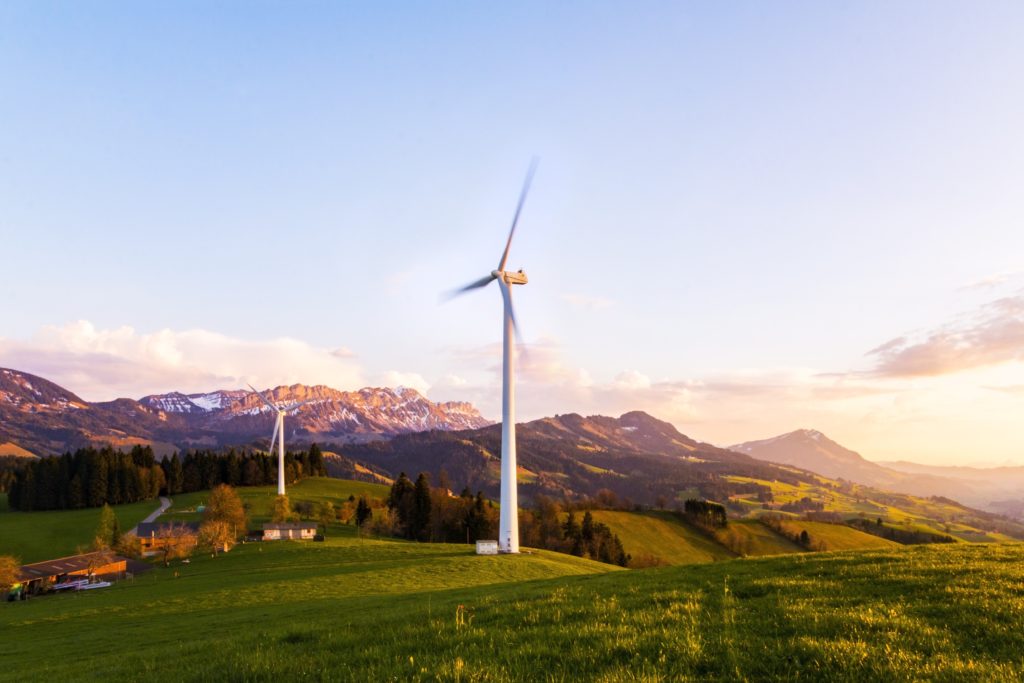
Research Ep 1
Net-Zero by 2050
Pledges to achieve “net-zero” emissions are proliferating from companies and countries alike. However sincere these commitments may be, they rarely include specific plans to achieve that ambition. The Net-Zero America Report from Princeton Universi
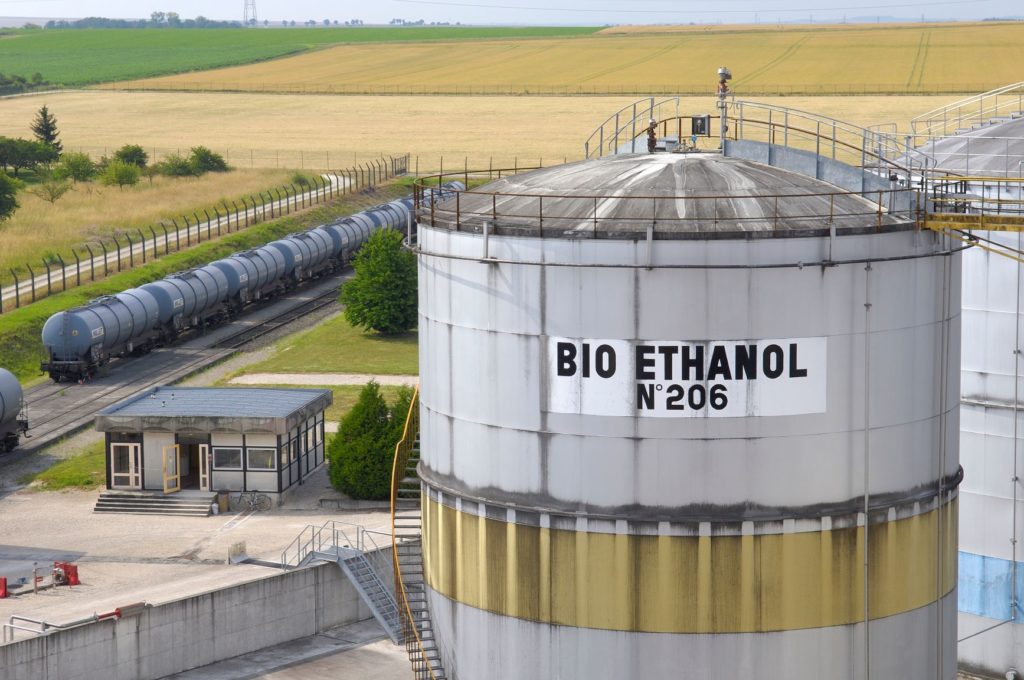
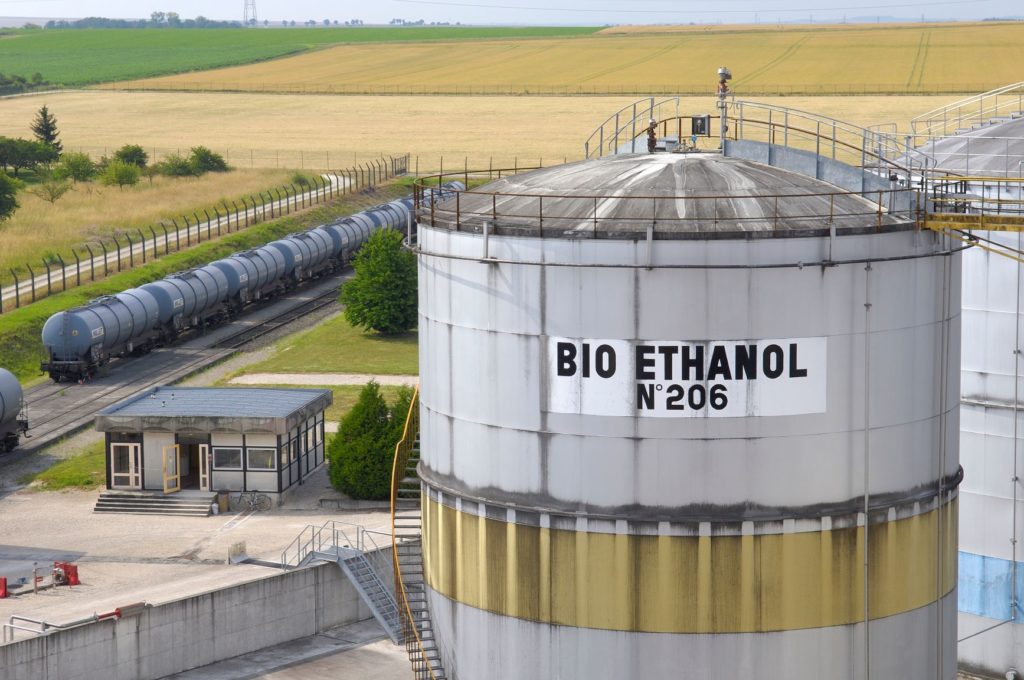
A Climate Change Primer Ep 6
Biofuels: An overview
Biomass – such as corn or switchgrass – can be converted into liquid transportation fuels, or biofuels. Biofuels are attractive because they result in significantly fewer emissions than fossil fuels, but they come with their own set of challenges,
Episode Transcript
Bioenergy Conversion with Jerry Tuskan TRANSCRIPT
Katherine Gorman (00:02):
You are listening to Climate Now. I’m Katherine Gorman.
James Lawler (00:05):
And I’m James Lawler.
Katherine Gorman (00:06):
And James, today we’re going to explore the idea of biofuels, which just always makes me think of like those vats of algae that are supposed to produce oil, like sort of science-fictiony, kind of out there, very expensive, but possible. What is it you think of?
James Lawler (00:25):
I think about corn. I think about corn, corn and ethanol, like large amounts of un-eatable, inedible corn, making gas additives. That’s what comes to mind.
Katherine Gorman (00:38):
And that’s something that we already experience, James. So you holding up your end of the bargain as the reasonable one, and me being the crazy one. I love that we play to our tropes.
James Lawler (00:49):
But there’s so much more that we don’t know about yet. In this episode, we are planning to break down the science behind biofuel technology and how biofuels might play a critical role in displacing fossil fuels from the energy market.
Katherine Gorman (01:05):
And to help us do that. We have Dr. Jerry Tuskan with us. Dr. Tuskan has been working with the Oak Ridge National Laboratory since 1990. And they’re currently the Chief Executive Officer of the Center for Bioenergy Innovation, as well as the group lead for plant systems biology in the biosciences division. He also holds a joint appointment at the DOE Joint Genome Institute in California, where he’s on the leadership team for the plant sciences program. So really an expert in all of this who can help us detangle these ideas. Dr. Tuskan, thank you so much for joining us. We’re really honored to have you with us for this conversation today.
Jerry Tuskan (01:41):
Well, it’s my pleasure to be here, and thanks for asking.
Katherine Gorman (01:44):
So, to start things off, we like to ask the same question first to all of our guests. How did you get where you are today in your career?
Jerry Tuskan (01:51):
You know, I grew up in Arizona and my dad and two brothers and I would go camping a lot. And so I really learned to love the outdoors and I decided as a high school senior to enter into forestry. And so I started my undergraduate education as a forestry major. And when I was a sophomore, I took a genetics class. From that point forward, I decided I was going to practice plant genetics. Went on to finish my degree, undergraduate degree in forestry. And I got a master’s in forest genetics from Mississippi State and then a PhD in genetics from Texas A & M. And my first job was at North Dakota State University where I was in the horticulture department. And then a job came open at Oak Ridge National Laboratory and I applied thinking I would work for five years, get some experience in a government lab, and go back to academia. But it’s been over 20 years now and I’m still here at ORNL. I’ve enjoyed it greatly. It’s provided me with wonderful opportunities in science.
Katherine Gorman (02:59):
Tell us about your work at Oak Ridge national laboratory.
Jerry Tuskan (03:02):
I’m the director of the Center for Bioenergy Innovation, which is a multi-institutional center. There’s 17 partners distributed across the US. We’re funded by the US Department of Energy, the office of Biological and Environmental Research. And we’re one of the four bioenergy research centers in the US, and our charge or our mission is to develop alternative fuels to displace petroleum-based liquid transportation fuels. And there’s about 265 scientists in the Center here at ORNL distributed at our partners institutions as well. And we cover everything from plant sciences to microbial genetics, to techno-economic analysis.
Katherine Gorman (03:48):
So, let’s start off with the basics, the very basics. Can you define what a biofuel is for us?
Jerry Tuskan (03:54):
Oh yeah. Good question. A biofuel is liquid fuel derived from biomass. And biomass is just dried plant matter. So imagine taking wood chips or chopped up grass and converting it, using a bacteria, into a liquid transportation fuel, and that becomes a biofuel. It can take the form of a gasoline drop-in product, or it can be a replacement for gasoline, diesel, or aviation fuels.
Katherine Gorman (04:27):
So the biofuels that are available commercially today, what are they being used for?
Jerry Tuskan (04:32):
So there is first-generation biofuels available today to consumers. Most people don’t recognize it, but the ethylene that they see in their gas pumps during the winter, and particularly the ethanol blended gasoline is mostly derived from biofuels. And so that’s between 10% and 15% drop-in fuel, on a weight basis. Those are first-generation biofuels. They come mostly from starch and out of corn kernels, but what we’re working on are lignocellulosic second-generation biofuels. Lignocellulosics are really comprised of three polymers. Lignin, cellulose, and hemicellulose. And these are the components that make up the plant cell wall. Plants are rigid. You know, when you try to bend a plant, particularly a woody plant, it’s hard. And it’s because the cells are layered with cellulose, which is a glucose polymer; hemicellulose, which is a five-carbon sugar polymer; and lignin, which is a phenolic polymer. And these intertwine into a network that envelops the plant cell. We’re trying to use enzymes derived from bacteria to deconstruct those cell walls and release the sugars so that they can be fermented into fuel.
James Lawler (05:57):
So before diving into the technology that you’re developing, I’d love to ask you why are biofuels important for us to focus on at this moment?
Jerry Tuskan (06:07):
Biofuels give us the opportunity to displace petroleum-based transportation fuels. Oil or coal represents carbon that was fixed a long time ago, and that’s ancient carbon that’s been captured and held in the ground for a long period of time. Because we use internal combustion engines, that carbon is burned and admitted back into the atmosphere in the form of CO2. So by using biofuels, we can displace that petroleum and reduce the amount of carbon dioxide emissions in the transportation sector here in the US. So it’s a means of reducing the amount of carbon through capture of growing biomass, Poplar, or switchgrass or other forms of plant biomass, harvesting the above-ground portion and converting that into fuel, and leaving the perennial root systems intact in the soil so that that carbon remains fixed in the soil. So we both get a displacement effect from the conversion of biomass into fuel, and a sequestration effect because of the carbon remaining in the soil, in the perennial root systems.
James Lawler (07:22):
Now, you’ve already touched on this a bit, but could you say a little bit more about the total lifecycle of CO2 emissions stemming from biofuels? Another way of posing this question is, are these fuels net-positive in terms of emissions or net negative?
Jerry Tuskan (07:38):
So first-generation biofuels in the form of ethanol that we use now in gasoline is CO2 neutral. It’s not positive or negative because corn, first-generation biofuels are produced from starch found in corn kernels, and corn is an annual crop that is planted and harvested annually. There is a net-zero benefit from using biofuels. It has advantages in terms of reduction of pollutants. We’re working on second-generation lignocellulosic biofuels, and this is where perennial plants come into play. So we can think about our agricultural landscape and converting, marginal agricultural land into these perennial systems of grasses and trees that sequester carbon in the soil, and then are harvested once a year and allowed to regrow. And so you have a very positive impact on CO2 using lignocellulosics. You displaced the petroleum – positive effect there – and then you have the sequestration of the carbon fixed in the root systems that remain intact over long periods of time.
Katherine Gorman (08:54):
Can you walk us through the steps of biofuel production, starting from bioenergy crops and ending with usable fuels.
Jerry Tuskan (09:02):
The process of creating biofuels in our center starts with growing improved plant materials, Poplar, and switchgrass. You establish those in a field much as you would any other agricultural crop, but we’re targeting marginal agricultural land to avoid competition with food production. So this will go, we’re looking at areas in the Western great plains or abandoned agricultural land in the Southeast or the Northeast. Poplar and switchgrass would then be planted and grown over multiple periods of time, harvested, and brought into a conversion facility. In this conversion facility, there would be a bio-reactor and the bio-reactor would contain bacteria, a bacteria that’s been engineered to recognize this biomass so that the plant material itself, although is difficult to deconstruct (that’s a term called recalcitrance it’s recalcitrance), this bacteria has been programmed to recognize that and the plant cell walls have been modified to match the bacteria so that it easily deconstructs these polymers. So lignin is stripped away, then the hemicelluloses and celluloses are de-polymerized, broken down into their basic components, which is glucose or five-carbon sugars like fructose. The glucose has been fermented, you know, much the same way that we think about making ethanol for human consumption, fermentation takes place. But this is where the process differs. Instead of distilling that alcohol into a consumable product, we take the ethanol vapors right out of the bio-reactor and the ethanol vapors are passed across the catalytic bed. And that catalyst converts the ethanol into a hydrocarbon. And that hybrid hydrocarbon has properties similar to aviation fuel or diesel fuel. So it can be used as a replacement for petroleum-based aviation fuel. And so it’s the combination of improved feedstocks, biological deconstruction and fermentation, and catalytic upgrading that leads to the second-generation biofuels.
James Lawler (11:31):
When we talk about the chemistry in this process, focus us on, you know, where does the energy come from?
Jerry Tuskan (11:39):
I can talk in generalities in the sense that, lignin is a high energy compounds because it has, um, quite a few carbons per unit weight relative to other biological materials. Cellulose interestingly enough is the most abundant polymer on the planet. There’s more cellulose than there is any other polymer on the planet. And it contains energy captured in the molecules of glucose. Those molecules are comprised of carbon, hydrogen, and oxygen that makes up the glucose, but for a hydrocarbon fuel, you need to remove all the oxygen. And so that’s the purpose of the catalytic upgrading, is it scours out all the oxygen and just leaves the hydrocarbon, a high energy, energy dense, liquid fuel,
James Lawler (12:37):
And what qualifies as good feedstock. Why focus on plants like eucalyptus, switchgrass, and Poplar, for example?
Jerry Tuskan (12:45):
So the US Department of Energy, 20 years ago published a report that evaluated all possible sources of biomass, including agricultural residuals and wastes, forestry residuals, construction materials, and dedicated energy crops. And that portfolio was the first indication that we have enough biomass, that publication was the first indication that we have enough biomass to actually displace transportation fuels. Of all of those sources, dedicated energy crops include things like Poplar, switchgrass, eucalyptus, willow, Miscanthus, energy cane, and these plant materials have many things in common. They’re typically fast-growing. They typically can grow on that marginal agricultural land, so they don’t compete with food. And they have the benefit of most of them being perennials. And so we chose to work on Poplar and switchgrass, not because the others were more or less suitable, but that the genetic systems in those two organisms allowed us to select for improved cell wall traits, which is where the biomass holds the energy, in its cell walls, in its lignin, cellulose, and hemicellulose.
Katherine Gorman (14:17):
So I’d love to talk a little bit about air travel and aviation fuel. I think it’s often a topic that gets brought up in this conversation and people just end up throwing their hands up about it, but why is aviation fuel or jet fuel so difficult to produce alternatives to, I mean, isn’t it just an engine? Does it, does the fuel have to work in some kind of a different way? What makes it so difficult?
Jerry Tuskan (14:43):
What makes aviation fuel special? Well, they have very tight technical requirements that we all appreciate. If we decide to fly from LA to New York, we’re in mid-air for five hours, we want the engines to function properly of course, and that requirement dictates that aviation fuels have a series of parameters that determine its suitability, things like flashpoint, freezing point. Aviation fuel in a jet engine also acts as the lubricant. In our engines and our cars we put in gasoline, but we also put in oil, and the oil lubricates the engines, but in jet engines, they act as the coolant, as the lubricant, and as the energy source. So the requirements are very tightly controlled and specified. It makes producing them from biomass difficult. And we’ve developed a catalytic upgrading approach that allows us to create these hydrocarbons that have the same properties as jet fuel, olefins and aromatics that are found inside this combination of chemicals known as jet fuel. And so once that happens, once we’re able to produce a biofuel in the form of an aviation fuel, it has to be tested and certified. And we’re in the early, early stages of that testing and that certification.
James Lawler (16:23):
And what about the enterprise of developing jet fuel? How did, how do new fuels get certified? How do we sort of ensure that they are safe and, you know, capable of working as a drop-in fuel?
Jerry Tuskan (16:38):
So there are multiple levels of certification and the first level is tier zero or level zero, where you’ve created what you believe is a jet fuel, a biomass-based jet fuel, and we’re producing in this case, hundreds of liters, a small volume, that then can be tested chemically. Does it have all the chemical properties, does it behave under high temperature, or low temperature? And that is before it even is placed into a jet engine. And from that point forward, we have to partner with organizations that do the certification. We can’t certify it ourselves. So we partner with organizations like CAFFI, Steve Csonka is the president of CAFFI, which is an organization that helps promote sustainable aviation fuel, and then links us to laboratories around the country that do the certification all the way up to jet flight, not just jet engines, but jet flight testing without passengers, of course. And that requires hundreds of gallons of fuel. So we’re at the dozens of liters in volume at this point, but we have to scale that up, which means you need larger bio-reactors. I talked a moment ago about bacteria inside a bio-reactor and us pulling the alcohol vapors off that bio-reactor and upgrading it, that that is a two liter reactor, but in an industrial complex, you need a 500 liter or a 5,000 liter reactor. And so of course there are industrial complexes that have these, but they’re not available within the center here. So we have to partner with these scale up or pilot facilities and provide them with the biomass so that they can produce enough liquid fuel in the form of aviation fuel to test in tier two, three and four.
James Lawler (18:58):
I’d love to sort of go a little bit further upstream in the biofuels production process. Can you describe how biomass feedstock is broken down via this special bacteria that you talked about earlier and turned into a fuel?
Jerry Tuskan (19:14):
Yeah, this process has to be tightly coordinated. In addition to just the feedstock, which would be the Poplar chips or the switchgrass straw, you have to have the right bacteria in the bioreactor. The bacteria we use is Clostridium thermocellum, which is a thermophilic anaerobic bacteria. So it lives at high temperature in the absence of oxygen. So the bio-reactor has to be sealed and has to be capable of generating enough heat that the Clostridium deconstructs and ferments the biomass. In addition to that, we apply a process called co-treatment. And here you provide, let’s say, Poplar chips, the size of a potato chip, 500 pounds of those, to this bio-reactor, in the presence of the bacteria, the biomass becomes softer. It, the bacteria overcomes the recalcitrance. You could let that process take place over seven days and ultimately the bacteria would digest and consume all the biomass and form alcohol, but we can accelerate that process by co-treatment. It’s brief milling in the middle of the deconstruction process. So we can go from a seven day process to a two day process. There are three parameters associated with producing liquid transportation fuels in a bio-reactor. They’re tighter, rate, and yield. And so you want to have tighter as high as possible, rate as fast as possible, And yield as high as possible. Tighter is just the amount of fuel that’s contained in the bio-reactor after deconstruction and after fermentation. So there’s a negative inhibition or feedback loop that happens as bacteria produce more alcohol, they inhibit alcohol production. So we had to modify the bacteria to tolerate higher and higher concentrations of alcohol to get tighter high enough to be economically viable. Rate is the speed at which the deconstruction fermentation process happens. We’ve been able to reduce the fermentation times from seven days down to two days, which becomes economically feasible. And then yield is the amount of carbon you get into the form of fuel that was derived or delivered in the form of biomass. And so you want to achieve as high a yield as possible, 90% or more of the carbon going from biomass into fuel. And so those three parameters have dictated how we design the bio-reactor and how we process the biomass. And so we want to have the biomass in a form that’s easily deconstructed to get maximum yield, we want to have the rate as quick as possible, so we use this process called co-treatment, which is brief milling in the middle of deconstruction and fermentation, and then we modify the bacteria, the biological agents, to tolerate higher and higher concentrations of alcohol. And this drives us towards an economic point where we can compete with aviation fuel from petroleum-based sources.
Katherine Gorman (23:02):
So, touching on that point about competing with fossil fuels, what’s the current price point of the technology you’re developing in terms of dollars per gallon of aviation fuel.
Jerry Tuskan (23:15):
So to be competitive with petroleum based fuels is a very complicated thing. It’s dynamic. The price of oil goes up or down depending on what’s happening in the world. And so, to hit a specific competitive threshold varies from month to month almost, but on the average, our target is to produce gasoline-like biofuels at $2 a gallon retail, $2.50 a gallon for aviation fuels.
Katherine Gorman (23:53):
That seems pretty cheap for aviation fuel doesn’t it?
Jerry Tuskan (23:53):
The price of aviation fuels fluctuates greatly and, depending on demand and supply of course, and to be competitive without externalities or government assistance, we think we need to hit as low a baseline cost as possible. Our initial estimates were about $6 a gallon. And so we’ve slowly begun to reduce that, we’re somewhere around $4 a gallon now, and we think we can get it to $2.50 a gallon.
Katherine Gorman (24:29):
What do you think it’s going to take to get us from that $4 per gallon of aviation biofuel to the $2.50 number per gallon?
Jerry Tuskan (24:39):
There are many, many factors that affect the cost of transportation fuel. Particularly those derived from biomass. The cost of the feedstock is about 50% of the cost of the fuel. And so anything you can do to reduce the cost of the feedstock helps. There the biggest driver is yield, the tons per acre of biomass you can produce. Our target is 10 dry tons per acre, per year productivity. And so that encompasses areas of cold weather and hot weather, dry seasons and wet seasons, various soil types, various insects and diseases. And so the challenge is to create a robust, sustainable feedstock that can be deployed on 20 million acres of land, achieve 10 dry tons per acre per year, and costs somewhere around $50 a ton to deliver to the mill. Once the biomass is delivered to the mill, and you want to process it as efficiently as possible, I’ve already talked about tighter, rate, and yield, and so you want to maximize tighter, reduce the time it takes to convert the biomass, and then increase the yield. So we’re working to achieve those things simultaneously, matching the bacteria to the feedstock so that they’re optimally connected, that deconstruction happens as effectively and as efficiently as possible, and then in terms of catalytic upgrading, you want to be able to use a catalyst that is cost-effective, that’s affordable. Many, many catalysts use rare earth elements that are very expensive. They are not reusable in an easy manner. And so we’ve decided to go with a single stage catalytic upgrading using zeolite catalyst that don’t contain rare earth elements. And we found the combination that does this very effectively. Now our challenge is to tune the product. We get an array of hydrocarbons from diesel to jet fuel in a mixture. And so we want to be able to tune the catalyst so that we can predictably produce just diesel fuel or just jet fuel. And that’s a challenge of chemistry to get the right combination of elements in the catalyst, so that it’s cost effective and affordable and predictively yields the right hydrocarbons.
James Lawler (27:34):
Dr. Tuskan, I’d love to turn to a paper that you shared with us, which is titled “Robust paths to net greenhouse gas mitigation and negative emissions via advanced biofuels.” Now, this paper contains a quote that says the “climate benefits of cellulosic biofuels have been challenged based on carbon debt, opportunity costs and indirect land use change, prompting calls for withdrawing support for research and development.” Can you talk to us about what those arguments are as well as the reality of the state of biofuels.
Jerry Tuskan (28:04):
The debate is going on, and there are three different elements to this debate. Placed in different terms, opportunity cost is what a landowner does with their land. You could decide I’m going to leave it fallow. And there’s an opportunity cost that you could have grown corn or soybeans and gotten profit from it. So you’ve missed that opportunity. The same thing applies if you decide to grow biofuels, there’s an opportunity cost of not producing corn or soybeans. And so the return on investment for biofuels has to compete with existing land use, to return on the farmers investment in that land. We can’t compete with corn and soybeans. The return on investment to the land owner is so high. So we have to find opportunities where bioenergy could compete and provide a greater return on investment to the landowner. We’ve talked about optimizing land use in terms of opportunity cost. There’s also the issue of competing with food production. So we target these marginal agricultural lands that I talked about earlier. Then there’s the argument that you see in the literature about net carbon impacts on atmospheric CO2, and many of those have focused on first-generation biofuels, derived from starch in corn kernels. Those tend to be neutral. So we have to come up with a system that’s net-positive in terms of carbon capture, carbon recycling, and carbon use in the form of fuel. And we’ve done that through the use of perennial, the deployment of perennial plants on the landscape. And I’ve mentioned that these root systems remain in the soil as a perennial carbon sink for many, many years, and you continually harvest just the above ground portion. And so there’s a net benefit there of advanced or second-generation biofuels. So I touched on all three of the criticisms, and then just would summarize by saying that our techno-economic analyses indicate that advanced-generation biofuels from lignocellulosic feedstocks provide a net carbon benefit, provide the right opportunities for landowners to get a return on investment, and don’t compete directly with displacing food production.
James Lawler (30:54):
And that’s because we’re talking about targeting marginal land, is that right?
Jerry Tuskan (30:57):
We’re targeting marginal agricultural lands. There’s about 420 million acres of agricultural land in the us, and we would target 20 million acres in the Midwest, but the Western Midwest, areas in Western Kansas, Western, Nebraska, South Dakota, North Dakota, which are not highly productive agricultural lands.
James Lawler (31:22):
Got it. And where would you say the opportunities are for deployment of capital? Say for large investors who are interested in a longer term horizon?
Jerry Tuskan (31:32):
The capital investment in a biofuels facility is measurable. It would take millions of dollars to create a 5,000 gallon-a-day bio-refinery. These are large, large facilities that your average investor is, would not be capable of making. It will take a combination of government insurance and capital investment to make this happen. It will not be feasible without large investments from both the private sector and from government.
Katherine Gorman (32:13):
Great. Jerry, thank you so much for your insight into this topic and for taking the time to join us today, really appreciate it.
Jerry Tuskan (32:25):
Yeah.
Katherine Gorman (32:25):
That is it for this episode of the Climate Now podcast. You can check out our other interviews, watch our videos, and sign up for our newsletter on our website, climatenow.com. If you want to get in touch, you can email us at contact@climatenow.com or tweet at us, @weareclimatenow. We hope you’ll join us for our next conversation.