Featured Experts
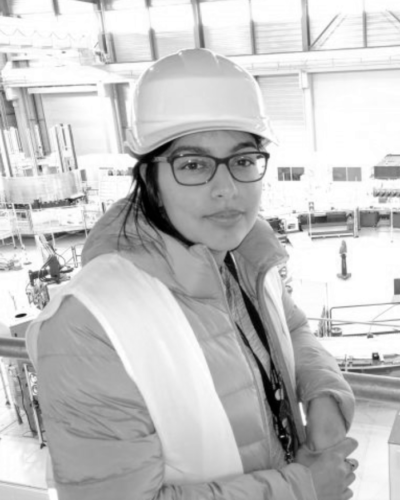
Aneeqa Khan
Research Fellow in Nuclear Fusion
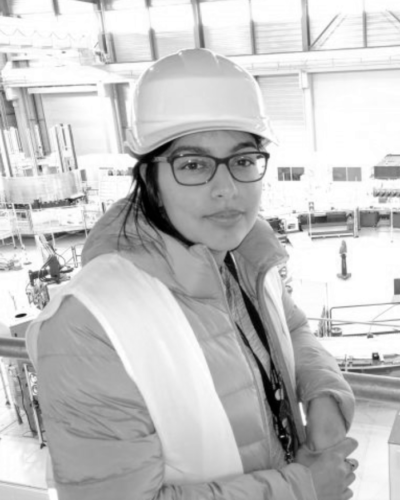
Aneeqa Khan
Research Fellow in Nuclear Fusion
Aneeqa Khan is a Research Fellow in Nuclear Fusion in the Department of Mechanical, Aerospace, and Civil Engineering (MACE) at the University of Manchester. Her research is focused on testing materials and components for nuclear fusion applications. She previously was a postdoctoral scholar at the international nuclear fusion project ITER.
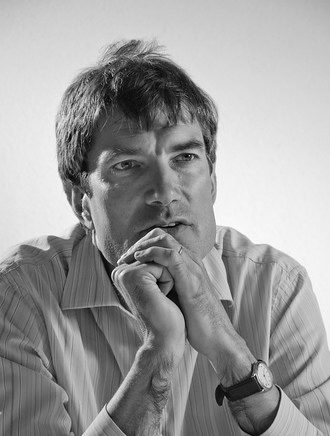
Steven Cowley
Director of the Princeton Plasma Physics Laboratory
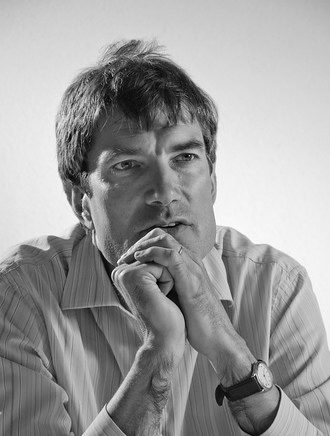
Steven Cowley
Director of the Princeton Plasma Physics Laboratory
Sir Steven Cowley is a theoretical physicist and international authority on fusion energy. He is the Director of the Princeton Plasma Physics Laboratory (PPPL), and a Princeton professor of astrophysical sciences. He was most recently president of Corpus Christi College and professor of physics at the University of Oxford. Cowley previously was chief executive officer of the United Kingdom Atomic Energy Authority (UKAEA) and head of the Culham Centre for Fusion Energy.
In this Episode
Is there such a thing as “perfect” energy? With nuclear fusion, the answer is maybe.
Fusion energy would be safe to human health, environmentally clean, and essentially limitless.
But, developing a sustainable fusion reaction still faces significant engineering hurdles and is likely decades away from becoming a reality.
So, where are we in the development of fusion technology? What technical challenges remain? And what practical challenges must be overcome to make fusion a competitive energy source?
Sir Steven Cowley, director of the Princeton Plasma Physics Laboratory, and Dr. Aneeqa Khan, Research Fellow in Nuclear Fusion at The University of Manchester, answer these questions in this episode.
Related Media:
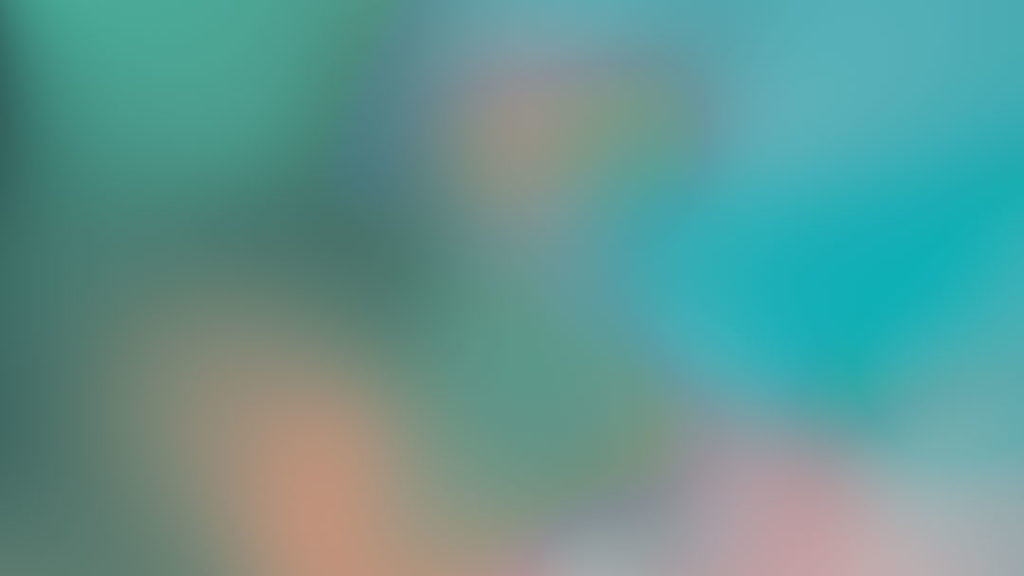
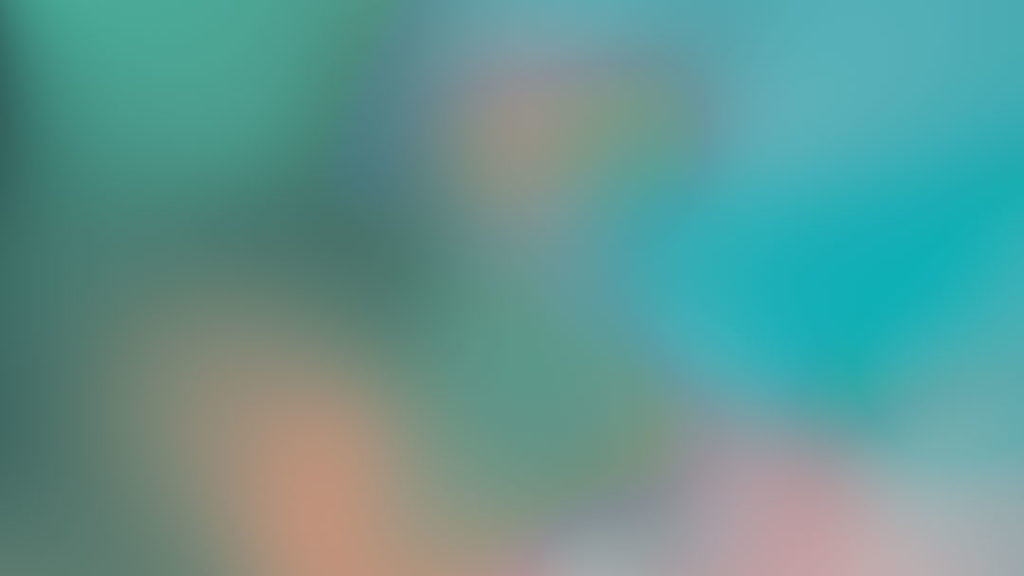
Climate Now: Aug 27, 2021
Nuclear Energy: What are the real risks? with David Keith
Despite being a reliable, zero-emissions alternative to energy derived from fossil fuels, nuclear energy remains mired in controversy. Opponents often cite four reasons not to include nuclear in the portfolio of alternative energy sources that will replace fos
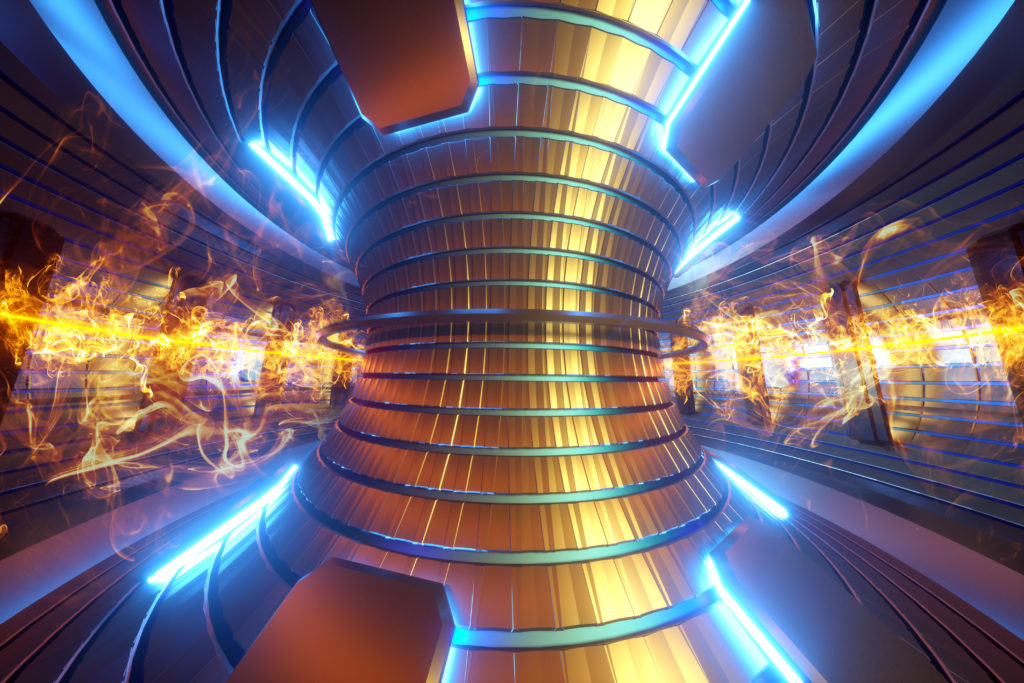
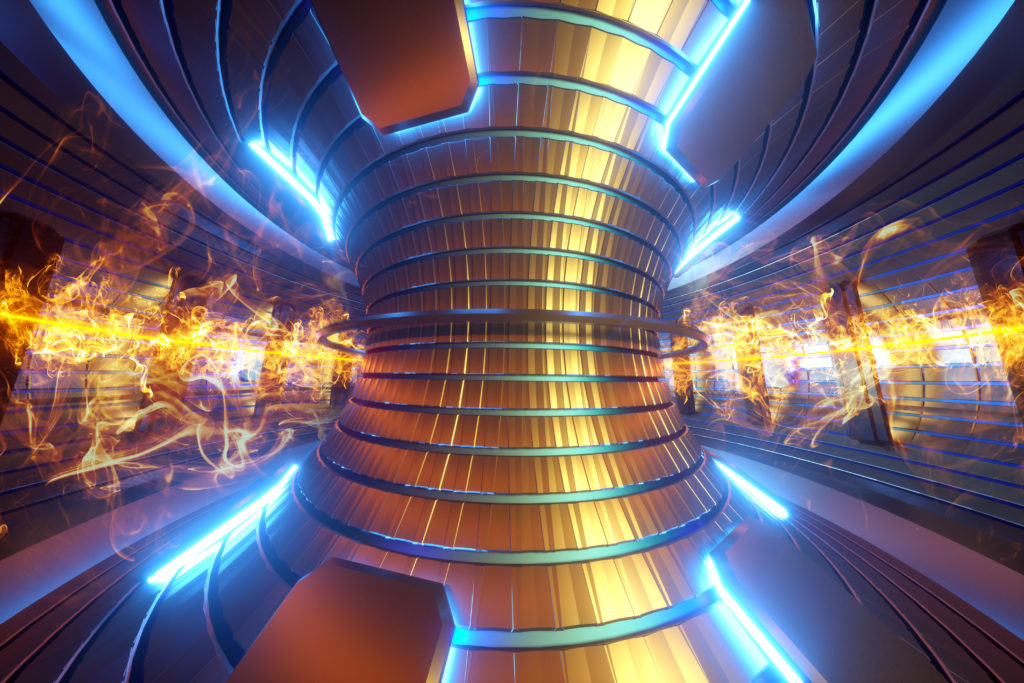
Technologies Ep 3
Building stars on Earth: the potential of nuclear fusion
Energy from nuclear fusion has the potential to cleanly and safely power the world. But, when do fusion experts expect this to happen? What technical challenges must be overcome before we can power our homes using fusion energy? Which technologies are lead
Episode Transcript
PODCAST TRANSCRIPT:
Katherine Gorman: You are listening to Climate Now. I’m Katherine Gorman.
James Lawler: And I’m James Lawler.
KG: And today we are going to talk about a developing technology – actually perhaps the most challenging technology in terms of how difficult it will be to achieve, and significant with respect to the impact it could have on our energy sector, and that is nuclear fusion.
JL: Yes. We set out to understand both the fundamentals of how fusion works, and to get some idea about how and when it might enter into productive use to power the planet. This episode combines conversations with two experts in the field. We began by speaking with Sir Steven Cowley, a theoretical physicist and international authority on fusion energy. Dr. Cowley currently directs the Princeton Plasma Physics Laboratory at Princeton University, and was previously the chief executive officer of the United Kingdom Atomic Energy Authority and head of the Culham Centre for Fusion Energy in the UK. We also spoke with Dr. Aneeqa Khan, a mechanical engineer and Research Fellow in Nuclear Fusion at the University of Manchester, whose research is focused on testing the materials and components for nuclear fusion applications.
KG: Dr. Cowley, thank you so much for talking with us. Now, I would like to start with the basics. What I understand about nuclear fusion is that it is a process where we are mimicking what is happening inside a star, right? Like, the process that makes our sun give off heat and light, is nuclear fusion?
Steve Cowley: So inside stars, what stars are doing is this kind of remarkable process, they’re taking hydrogen and they’re putting it together and they’re making helium, and they do this by a process called nuclear fusion. And then they take helium and they put it together and they make carbon. And then they add on to that and they make all the elements that are in your body. And it’s an interesting thing, but we’re all in fact Stardust, right? Everything in your body, somehow at some point in time, went through a star and all the elements that are in your body were created by this process of fusion, which is sticking together small nuclei, fusing them and making bigger nuclei out of it. And when you do that, you release lots of energy, a tremendous amount of energy for it each time. And that’s why the sun is hot, right? That’s why stars shine. And so fusion, in some sense, is the thing that makes life happen. So the real question for a scientist is could you ever tap that source of energy? Could you use fusing small nuclei to make bigger nuclei as something that could power the planet?
JL: And so this nuclear fusion, this is very different than nuclear power that is generated in modern day power plants, which is nuclear fission. Can you walk us through the distinction between those two types of nuclear energy generation?
SC: So nuclear fission is what we do in conventional nuclear power stations. And what happens there is uranium, which is an enormous nucleus with lots and lots of particles in the nucleus, splits into roughly two nuclei about half the size, and that’s called fission. Because it fissures down the middle, and then it splits up. So fusion is that process that makes nuclei bigger and fission is that process that makes them smaller. So explain a bit more about fusion, right? So there are these two forces, right? There’s the strong force, which glues the nucleus together, and then there’s the electrostatic force, the force of electricity, which, because nuclei are charged, positive charges, they repel each other because of that and distance, they always repel each other. And when you get closer and closer and closer to each other, they will start to attract. And when you’re very close, they’ll attract very strongly. Right? And so what you’ve got to do to get fusion to happen is you’ve got to get the two nuclei really, really close together – about 10 to the -14 meters. An incredibly short distance. And to do that, you’ve got to get over the fact that they repel each other all the way until you get that close.
So the only way we know how to do that is to heat these nuclei up to very hot temperatures so that they’re running around really fast. And every now and again, they’ll bump into each other really hard and fuse. If they’re not moving fast enough, they’ll just bounce off each other. So you have to get the temperature, which is essentially the kinetic energy of the objects up to a temperature of about 200 million degrees. And at that temperature, you can get fusion in what we call hydrogen isotopes, heavy hydrogen, which is called deuterium and super heavy hydrogen, which is called tritium will fuse at a temperature of about 200 million degrees. Now, the problem is how do you hold 200 million degrees, right? You can’t have a bottle that holds 200 million degrees cause it’ll melt. And so the way we do that is that we make a cage of magnetic field and we hold the fusion fuel in a cage, of magnetic field.
And because it’s so hot, it’s no longer a solid or a gas or a liquid, right? It’s so hot that it’s, what’s called a plasma, which means that the nucleus of these atoms and the electrons are stripped off each other. And it’s called an ionized gas is another name for it. And then the magnetic field can push on it. And what you do is you basically push on the plasma of the fusion fuel and push it off the wall. So it doesn’t touch the walls. And then you heat it to 200 million degrees. And then it will fuse. And here at Princeton in 1994, we did that with fusion fuel for the first time. And we got 10 million Watts of fusion power coming out of a caged plasma.
JL: And what was that like when that happened?
SC: Complete exhilaration. I mean, you don’t do experiments like this without carefully planning up to it, but when it actually happens, you know, it’s amazing.
KG: Wow, so nuclear fusion has been done?!? We have created this in a lab. Does that mean that this is already a viable energy source?
SC: Well, so the big problem that we are still struggling with is that the cage of magnetic fields does not hold it, you know, very firmly. And in fact it can be leaky. And so if heat leaks out, then you’ve got to supply more heat to keep it hot. Eventually, we are building a machine in Southern France called ITER. It’s a massive international experiment. If you get the cage good enough, then fusion will heat itself enough that it will keep itself hot. It’s a bit like lighting a fire, right? When you put the match on the fire, you’ll get some wood to burn, even if it hasn’t really caught the light, but if it starts to generate enough heat to keep itself alive, then you know, you’ve got a fire. And that bit of fusion we haven’t done yet. The experiments at Princeton didn’t have a secure enough cage to keep the fusion fuel from cooling by leakage of the heat, to the walls.
One of the great things we have now that we didn’t have when I came into the field is that we can simulate on the computer, a fusion reactor, and therefore we can do the optimization without having to build very expensive pieces of equipment, and at Princeton right now, we’re trying to get an optimized configuration for a fusion reactor by just simply running an enormous amount of computer simulations to find the optimum point and optimize it. This way we can bring down the cost of getting to a sufficiently well contained fusion plasma that we can make electricity from.
JL: Well, so if our goal is to make electricity, we want to be putting in less energy than we are getting out of the system, right? From what you’ve described, you know, to initiate the reaction, you need to apply temperatures of hundreds of millions of degrees and you need to create this magnetic field to hold it all in place – doesn’t that cost a tremendous amount of energy? I mean, how could we get out more than we are putting in?
SC: Yes, well, the machine we’re building in Southern France, called ITER, which is an international machine, that is supposed to get to the point where you don’t have to put any energy into the plasma because it’s self-heated because the fusion heats the plasma and you’ve lit the fire, and you can take the match away. And that, I mean, when that happens, that’ll be amazing because then, you know, you can turn off all external heating sources and the thing will just burn, like a start. That will be, you know, one of those historic moments in science. And I’d like to be there since I have a little skin in the game.
JL: Amazing. And how close are we to that moment, do you think, and what stands between this, where we are now and achieving that at ITER?
SC: Well, so, you know, ITER is an international experiment. It’s supposed to start at the end of 2025, beginning of 2026, and I think it’ll take us several years to work up to that. You don’t, you don’t turn on an experiment, that’s cost you the best part of $20 billion, and just turn all the knobs up and say, right, let’s see if it works right. I mean, you slowly inch everything up to get there. Um, and that might, that will be a scientific demonstration if it works, a scientific demonstration that fusion can produce energy. It isn’t a demonstration that it can produce energy at a cost you want to pay for your electricity, unfortunately. But I’m hoping, and there’s a lot of political will right now to push forward on trying to do the innovation that’s necessary to produce cost effective electricity from fusion simultaneously, while we complete experiments on ITER.
KG: So this experiment at ITER, you put a price tag of almost 20 billion dollars on this. Wow. I think to understand what is coming out of that kind of investment, it would help to get a sense of what it is that we are actually making here. Can you describe for us, what is the physical expression of fusion – that is, what do the machines, or what do the reactors, look like?
SC: So, there are two really successful devices, fusion configurations. And the one that has led for several decades is called the Tokamak. It’s a Russian acronym for toroidal, which means donut-shaped device. And it was invented by the Russian dissident Andre Sakharov. Sakharov and his thesis advisor at the time were working on the Russian bomb. And they came up with this configuration and it’s the one that has brought us fusion. I mean, both the fusion here at Princeton and the fusion at JET in Europe, the lab I used to run in the UK, both those devices were Tokamaks. Now the thing about confining in a magnetic bottle is that magnetic field is basically lines in space and the particles follow those lines. And if those lines stay confined, then the plasma will stay confined.
But the problem is that lines have no ends. So essentially the lines have to go round and round and round and round and round. So you have to have something in which the lines can have no end. And as you can see, that means you need something sort of donut shaped. And the Tokamak, is a donut shaped thing, it has these magnetic field lines that go round and round and round and round around inside the thing and the nuclei follow that like with little spirals around those magnetic field lines going inside the device. And so the Tokamak has got us to where we are, but there’s another idea that came from the founder of this lab, Princeton lab called the Stellarator star maker, right? The Stellarator, unlike the Tokamak does not carry any electrical current. The Tokamak that we’re building in France has 15 million amps of electricity flowing around that loop. And that causes some technical problems that may not be optimal for a reactor. And so with the computer, we started to optimize Stellarators because they don’t carry any current at all. And it may be that this in fact is the one that’s going to sort of get us to real electricity from fusion, because the optimization on the computer really suggests that there’s something really there that we could really, make cost-effective electricity producing fusion from. So it turns out you have to get the magnetic field lines to twist it as well as go around the loop to confine the plasma. Now in a Tokamak, that twist is provided by the field produced by the electrical current, inside the plasma. So there’s a loop of electrical current going around 15 million amps, and that causes the field to have a twist in it. In the Stellarator, you don’t have that current, So we make the twist by kind of corrugating the outside of the Tokamak. So it’s no longer actually symmetric, you know, rotationally symmetric, it’s like a dimpled donut, right, where you kind of twisted the outside of the donut. And if we do that by having coils and magnets on the outside that twist it, you can get the same effect, but without having to have a current in the plasma. And so Stellarators look like something, I mean, they kind of look like very space age because the plasma is not, it doesn’t seem to have any particular shape whatsoever. It’s just sort of bent and twisted. And we could only really do this once we got the supercomputer. So it’s something really that we could only have done in the last 25 or so years.
JL: We have links on our website climatenow.com showing the Tokamak and the Stellarator built at the Max Planck Institute for Plasma Physics in Germany in 2015. They are pretty amazing to behold.
KG: Yes – and huge! They take up entire rooms! You know what is so incredible about this technology is not just its potential, or all the theory and the modeling that has to go into optimizing conditions to make it work, it’s also the incredible feat of physically building a machine that can hold something that is 200 million degrees! This is an engineering marvel as much as it is a scientific one.
We wanted to explore how realistic it is to build one of these (or many of these) to power our homes and our lives in the future, so we spoke with Dr. Aneeqa Kahn, at the University of Manchester.
Dr. Kahn, thank you so much for talking with us.
Aneeqa Khan: Thank you for inviting me. I’m really excited.
Okay, so I’ll go through each of the challenges. Well, there’s many challenges, but there’s kind of a broad, kind of categorization of all these challenges as it were. So the fusion reaction is typically between deuterium and tritium. Well, that’s the one that we want to recreate on earth. That’s the one we’ve decided is going to be the most promising for generating energy in the future. So that’s what we’re focused on. That reaction generates very high energy neutrons and those neutrons can interact with the materials that make up the reactor. So that is one key aspect of research is looking at what is the effect of this radiation. Does that affect the material properties, the mechanical properties of those materials, and does that make them worse, in the reactor? I mean they can fail. So that’s one thing that we look at.
The other one is the interaction between the plasma. So that kind of soup of positive and negative particles that are floating about inside the reactor is an ionized gas called a plasma that can also interact with the walls of your reactor. It can put bubbles in your materials, blisters, and again, that can affect material performance. It could also lead to fuel retention, which is something we don’t want. We’re using things like tritium, and we don’t want that trapped within the materials of our reactor. So that’s another key kind of materials challenge area. Also, as a fusion community, we’ve decided we want reduced activation materials, so we don’t want to generate high amounts of radioactive waste. So as well as having these challenges of the neutrons and the plasma, we’ve added an extra boundary condition that we don’t want to generate radioactive waste.
So we’re limiting the actual materials we can use for those kinds of scenarios. Another big thing is if we’re talking about magnetic confinement, fusion is generating those magnetic fields to confine the plasma, they’re huge magnetic fields. So ITER, we’re talking about 13 Tesla. So if you compare that to a PET scanner in a hospital, which is one and a half Tesla, which is pretty huge anyway, we’re much higher than that. So having magnets that can generate those fields is a huge challenge. And there’s been a lot of groundbreaking research on that. So we’ve got some reactors using these supercooled superconducting magnets that can generate the required magnetic fields. So ITER being one of them, but really exciting things going forward too are high temperature superconducting magnets, which are not actually high temperature, they’re still around 77 Kelvin. I think something like that. So it’s still very cold, but higher than the other superconducting magnets. So yeah, creating the materials that can make those magnetic fields is huge, and the effect of the magnetic fields on the materials themselves is another one. So that’s just some of the challenges of many, but those are some big ones.
JL: And which of those do you focus on, or do you think about all of them?
AK: Yeah, so we need to look at how they all are effected together. Like combined, what is the combined effect of those kinds of difficult conditions? Oh, the big one I forgot was the high temperatures. Can’t believe I forgot that. The center of the plasma is 10 times hotter than the center of the sun. We’re talking a hundred million degrees in the center of, you know, of this device, obviously not obviously, but those heat loads are not on the walls of the reactor. We’re still talking around thousands of degrees by the time you get to the walls, but still very, very hot. So yeah, the combination of the high temperatures, the neutrons, the plasma all combined is, it’s a very hostile environment. So yeah, working on all of those together is, is really important.
KG: And your job, as I understand it, is to try to find the kinds of materials that can withstand these conditions, right, this hostile environment. And I’m wondering if you can tell me a little bit about the process of figuring that out. I mean, it costs billions of dollars to build one of these reactors, right – so you want to have a good idea that your materials won’t, for example, be burnt to a crisp when you start the fusion reaction. So, how do you test whether or not the building materials for these reactors are going to work?
AK: So we won’t know if something works until we put it in the reactor itself, right. So we are doing that in some circumstances. So JET, for example, which is based in the UK, that has an ITER-like wall. So they’re running experiments there, to show what the conditions will be at ITER on the materials that they’re using. But it’s not as simple as that. There’s dedicated test campaigns around the world, with people trying to mimic these conditions and qualify different components for operational use. So that uses a variety of techniques. So some people are putting materials in fission reactors to see how they perform in fission reactors. Some people are doing, like high flux, kind of thermal heat loads and just, yeah, firing them with heat to see how they respond to those high temperatures and running them in cycles as well, to see how the cyclic heat, because in a tokomak, it’s not constant, right. It’s pulsed. So if we’re doing these cyclic loads that also has an influence, so yeah, they’re doing high heat flux experiments. There’s people doing linear plasma device experiments where they fire plasmas at materials to see how they perform. And then combining all of this together, then they kind of narrow down what materials qualify, and what will eventually be used in future reactors. I should say modeling is a very big part of this, but I just don’t do a lot of modeling at the moment.
JL: So, in addition to the materials you have to create for these, you also mentioned these specialized super-magnets. The one with 13 times the strength of those found in medical devices. Can you tell us a little more about those? Like, what do they look like, and where do they fit into the reactor?
AK: So for ITER they have coils, so it’s literally coils of this material that they use and it is wound up. And so, yeah, there’s a kind of cool magnets, these new high temperature superconductors. I may be wrong on this, but I believe they have that kind of tapes that they can quilt together. And that allows it to be kind of a more compact compared to these larger supercooled magnets that are being used in ITER, for example, but they’re really massive. So you can go on the ITER YouTube and see the magnets that they’ve produced. And they’re just huge. And the fact that they’re, this, this always blows my mind that they’re super cool. So they are almost absolute zero. I think they’re about four degrees Kelvin is kind of the temperature to which they’re cooled.
So you’ve got the outside of the reactor these magnets. Then in the center, you’ve got the center of the sun. So you’re going from about 4 degrees, Kelvin to 150 million degrees in the space of a few meters. And there’s nowhere else in the universe that has such a high temperature gradient. And so that always blows my mind. Like there is nowhere else where you’re going from basically absolute zero to 10 times hotter than the center of the sun in the space of a few meters. So that puts it in perspective a bit, for me.
JL: Right, unbelievable. To think that we are trying to build materials that can withstand the largest temperature gradient in the universe.
KG: It’s incredible. You know, it’s almost hard to wrap your head around technology this advanced, and this novel. And, as we were listening to both of our guests, the questions I kept coming back to is there really a future for nuclear fusion in our energy arsenal? Like, is this going to happen or is it closer to science fiction?
JL: Exactly, it seems clear that the technology is possible, but we wanted to know if it is practical. So we asked Dr. Crowley and Dr. Kahn about the logistics of making this happen – what are the risks, what would it cost? And what is the timeline, to make nuclear fusion a source of energy for the planet?
KG: I’d like to start by thinking about safety – there is a lot of hesitancy around expanding traditional fission nuclear energy because of the health and environmental risks posed if there were ever a leak or a meltdown. Is that a concern for fusion? Can a fusion reactor melt down?
SC: The answer is, it can’t. And the reason is, nuclear reactors have all their fuel in the big box of the reactor. That’s the fuel for years usually, right? The total energy content of the uranium rods that are in a nuclear reactor is enormous. But in fusion, what we do is we have this great big donut-shaped thing, and we puff in a little fusion fuel, and the fusion happens, and then we’ll puff puff in some more fusion fuel. And the fusion happens. And the amount of energy in that fuel at any one time is tiny. So if it all fused, right, it wouldn’t break through the walls, it wouldn’t do anything. And it’s usually only a few tenths of a gram of fuel inside this big container at any one time. And this means that there is no possibility that it can run away. There’s no possibility that it can breakout, there’s no possibility that it can result in a catastrophic accident. Just one of the reasons people think, you know, fusion actually has these amazing properties. It’s safe, it’s clean, and it’s plentiful. And that pretty much makes it perfect energy.
JL: You are dealing with temperatures that, you know, 200 million degrees inside of this plasma. So if something breaks in the reactor, what happens to all of that heat? Wouldn’t that be, is that not a danger?
SC: So the net heat, because it’s a tiny, only a tiny bit of fuel, the net heat is enough to melt, you know, about a 10th of a millimeter of the wall, if it all deposited on a square meter of the wall.
JL: Oh, okay. Okay.
SC: So it can’t burst through the wall, right, there isn’t enough heat there.
KG: So are there dangers or risks that we should be concerned about? I mean, with starting sort of with physical safety first or…?
SC: Well, I mean any industrial plant will have dangers, right?
KG: So, like, papercuts, yes, absolutely.
SC: No, no. I mean, here, you’re making electricity and love vast quantities of it. So any power station, you have to be very careful with it. The fuel that we want to use for the first fusion reactors as heavy hydrogen, deuterium, and super heavy hydrogen, it’s called tritium, and the tritium is made in the fusion reactor from lithium and lithium is perfectly safe. And so is deuterium. And so is that helium that you produce at the end of the reaction. But the tritium that’s in the middle is a radioactive gas. And so you make it and consume it on the site. And what you have to be careful of is that you don’t make more than you need or release any of that, because that is a, hazard. It’s not a hazard, like some catastrophic thing could happen, but you have to make sure that you do it properly. Right. I don’t want you to go away with the impression that there are no hazards and that this is all plain sailing. You have to do it right. And when you do it right, then there should be a minimal risk. There’s no risk of the kind of meltdown that happened at Chernobyl or Fukushima.
KG: Both Dr. Cowley and Dr. Kahn were clear that the risks associated with fusion, were far, far lower than that with fission, as fuel was only put in the reactor in extremely small doses at a time, instead of all at once, and the particles forming from the reaction were not the kind of dangerous radioactive waste that results from fission. So I asked Dr. Kahn if perhaps it made more sense for most of our research focus to be on fusion, rather than fission. Perhaps we could replace fission entirely?
AK: Very good question. I don’t think we should be focusing on fusion instead of fission at all. I think let’s keep focusing on fission to keep nuclear energy capable, and the renewable stuff. We need that now. Fusion is not going to fix whatever problems we have now. We need technology that is ready and working now, which includes existing renewable technologies, and then also fission as well I think will play a key role in that. I think the role fusion could play is not fixing our issues at the moment. But if I just use the UK as an example, because that’s where I am at the moment, on a kind of a very windy day, we actually get around 50% of our energy, or electricity, I should say, from wind power on a very windy day. When it’s not windy that drops to around 10%. And at the moment what fills those gaps is gas. So then we need some kind of source, energy source, electricity source, that has a stable base load that is able to fill those gaps in the future. And my idea is that’s where fusion could fit in towards the latter half of the century is filling in the gaps where the renewables can’t provide as much as what we need on those days and kind of replace fossil fuels in that way. But that doesn’t mean that we shouldn’t be using nuclear fission or other renewable sources at all, we definitely should be using them.
JL: Dr. Kahn, you also mentioned something to us in an earlier conversation that I thought was very interesting, which is that policy is a very important thing to start to think about, to be ready for fusion when it does become available. Can you describe what you meant by that? Why should we even be thinking about that, given where we are with the technology today?
AK: So if you look at any industry, they have some kind of regulation, whether its traditional nuclear industry, or even fossil fuel industry. When you are in industry, they all have regulations, which they need to follow, but putting those things into place takes time, and fusion is a new industry. And I don’t think the regulations from the traditional nuclear industry necessarily are fully applicable to fusion because we have very different challenges, reactors are very different in terms of what they look like, so developing policy and regulations that is relevant to fusion is really important and really time consuming, even if the technology was working now, if those policies weren’t in place that regulation wasn’t in place that would really delay us getting fusion onto the grid. So I think while we are still working on the technological challenges in parallel, we also need to be looking at this side of things so that we’re ready when those technologies are ready. So we don’t waste time on, doing things in series rather than in parallel.
JL: What kind of regulations are we talking about, and why is that something that will be difficult to put in place, given that you don’t really have the same concerns with fusion, right?
AK: I mean, I think we would still have some waste, even though we want to use reduced activation materials, we’d have kind of low-level waste, I would assume on at least the first fusion reactors that we produce, because we haven’t created all of these materials that might not produce any waste at all. So I think there would be some kind of low level waste. But it’s not just the radioactive waste, it’s just any new industry. So even if, you know, look at the fashion industry or makeup industry or whatever, it’s like, there’s so many kind of regulations in place to make sure that the moisturizer you use doesn’t contain something harmful. It’s not something specific to nuclear. I think it’s just any kind of massive industry, whatever it is, requires, huge amounts of regulation for things to be carried out safely, basically.
JL: So even though we’ve had fusion reactors for over 25 years, it seems as though there remains a lot of work to be done, a lot of steps still to take to get fusion technology online. We asked Dr. Cowley for a top-line estimate on when we’ll see the first fusion reactor hooked up to an electrical grid and supplying electricity to people’s homes.
Dr. Cowley, what year do you think that happens?
SC: I think we need to do that before the middle of the century.
JL: Do you think we will do it before 2050? In the middle in the 2040s?
SC: I do, because I think what’s going to happen in the next few years is, and I’m really pleased to see that the secretary of energy is pushing to deploy a lot of carbon-free energy in this next four years and get moving on decarbonizing the American grid, that’s the right thing to be doing, but getting to net zero, getting a big bite out of it is not too hard. Getting to net zero is quite hard, and there’ve been all these studies that show that it can be done, but it is, it needs a lot of work. Deployment of the first electricity in fusion will be a blip on solving the climate crisis. So really the impact of fusion is in the second half of the century, but I think my line on this has always been, we have some transitional technologies that we can use to decarbonize, the first one being fission, right. I don’t know that we should do fission for centuries. But I think we might do it, you know, for 50 years or a hundred years while we transitioned to net zero and make, cause it is a zero carbon technology. The second one is carbon capture and storage. We really don’t want to do for, you know, decade after decade because we’re going to have to bury tera-tons of CO2. And we don’t have the places to do that. And, you know, I don’t think it’s the technology that we want to do forever. So into that environment at some point, fusion is absolutely needed, right. What we have to try and do is to get it well before it’s needed so that it can be deployed, um, and deployed in that time.
KG: You know, this is such an interesting technology to be thinking about, because it is still at such a nascent stage. Dr. Cowley also talked a bit about the people who are investing in this technology. There are a lot of private companies, and private ventures working to close the gap on affordability – making a fusion reactor that is cost-effective. But at this stage, he mentioned that the investment is much more about making history, about making a huge leap in scientific knowledge than it is about return on investment.
SC: I think quite a few of the investors are quite involved in these companies because the thrill of being involved in making the ultimate energy. So this is the energy source that gives us life. This is the way the universe makes the energy that warms the planet that, you know, makes things grow. And to actually tap it now, once we know how to do fusion, we’ll never not know. If it works, it will change everything about the energy industry.
KG: That’s it for this episode of the podcast. To learn more about nuclear fusion, please check out our video on the topic. To hear more podcasts, watch videos, or sign up for our newsletter to get the latest, visit ClimateNow.com. We hope you can join us for our next conversation.